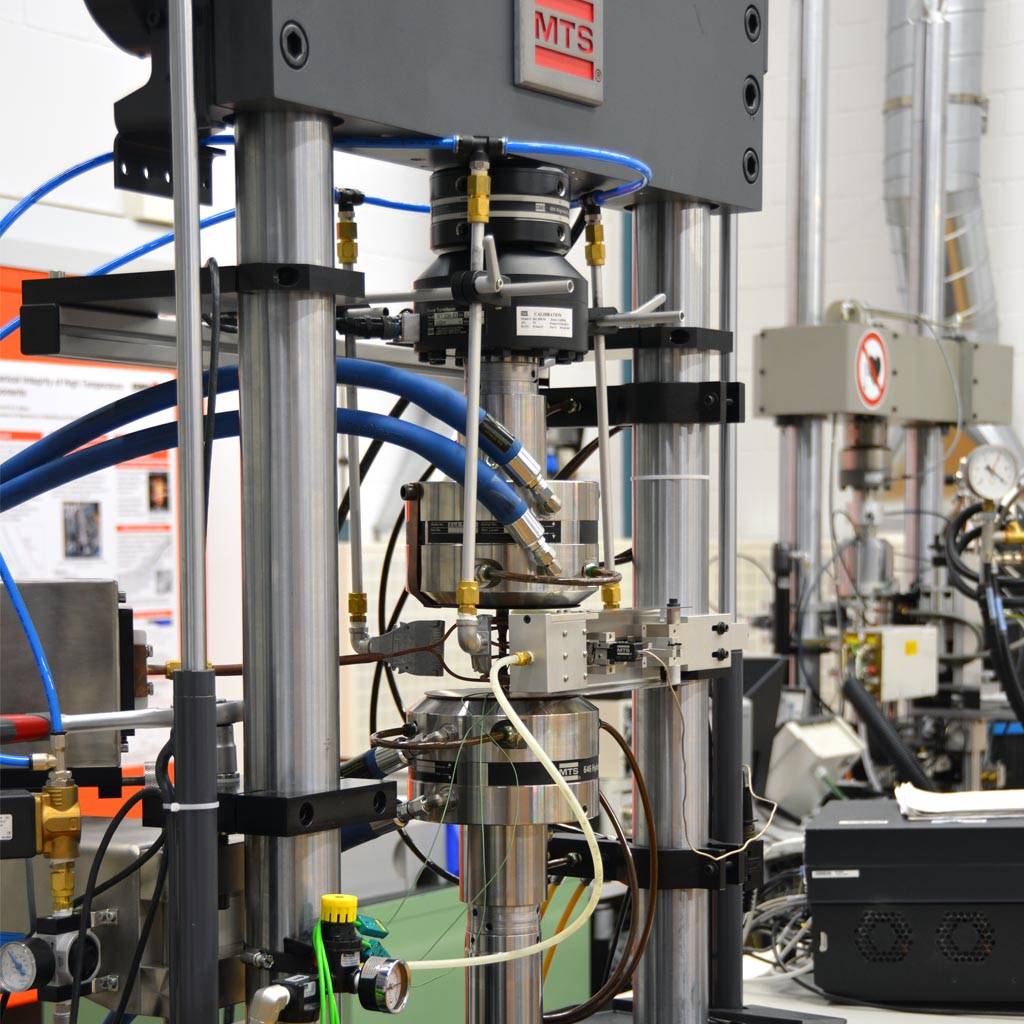
CUSTOMER CHALLENGE
Today’s gas and steam turbine designs are solving challenges that did not exist a few decades ago. Higher efficiency requires higher operating temperatures and materials that can resist oxidation and creep under these conditions. More flexible duty cycles mean these materials must also resist damage from thermomechanical fatigue. Of course, all of these improvements must be made without compromising operating costs or reliability.
To reach these goals, design philosophies are changing. As a result, conventional service life estimation methodologies are no longer always the best choice for accurately predicting the service life of turbine components. New methodologies are needed, but they must be tested and verified before manufacturers and end-users can trust them.
One lab that is pioneering developments in this field is Empa, a Swiss federal research institute located in Dübendorf, near Zurich. Using an innovative approach to thermomechanical fatigue (TMF) testing, Empa researchers are able to simulate close-to-actual turbine operating conditions with high fidelity and verify the effectiveness of new prediction methodologies.
Researchers use TMF technologies to effectively predict turbine service life.
“TMF testing has traditionally been used for material characterization,” said Dr. Stuart Holdsworth, Group Head of the High Temperature Integrity Group at Empa. “We are using the results of such tests to also form the basis of advanced material deformation and damage modeling concepts and, most importantly, for benchmarking the effectiveness of new high-temperature assessment procedures for predicting component lifetimes.”
Components within new turbines are designed to run as hot as possible — and thus as efficiently as possible — but not so hot that constituent materials exceed property limits. To avoid premature failure at high temperatures, it is necessary to fully account for time-dependent damage processes such as creep and oxidation, and their interaction effects on fatigue damage accumulation.
Simple creep or creep-fatigue studies focus on how materials react when controlled at steady high load and a high temperature. But this does not accurately reflect actual turbine service duty, in which loads and temperatures fluctuate.
“With the increasing tendency towards two-shift operation in the 1990s, and the subsequent increased adoption of combined cycle units, there has been an increased requirement for more flexible operation and the customer turbine start/stop number expectation,” Holdsworth said. “Today, the requirements are even more demanding.”
In the same way, duty cycles for jet engines are changing. Engines used in short-haul and regional aircraft experience shorter times between takeoff and touchdown. The shorter wings and narrower bodies of these aircraft mean that the engines encounter much different vibratory stress conditions than engines in larger, long-haul aircraft.
Because of this ever-changing array of duty cycles, it is difficult for turbine manufacturers to confidently predict how long each turbine component will last. Knowing the “weakest link” allows manufacturers to optimize new designs for reliability, and establish efficient maintenance schedules that focus on potential points of vulnerability.
This is why Holdsworth and his team devised an innovative approach to high temperature turbine component assessment, involving nonlinear finite element analysis and creep-fatigue damage analysis, as well as an effective means to benchmark and verify predictions.
The analysis technique follows an iterative process, starting with an initial creep fatigue assessment of specific turbine components. Advanced nonlinear finite element analysis is used to determine stress/strain states and critical locations in these components during service duty. Based on this information, the team can then make predictions about the component’s life span.
To determine the accuracy of these predictions, Holdsworth’s team developed a TMF testing methodology that can replicate the service cycle with the same thermal and mechanical stress/strain states that were identified in the initial analysis on the actual component. This process is then repeated, with results used to refine the analysis procedure until it is verified to predict service life accurately.
MTS SOLUTION
To perform its tests, the Empa laboratory uses two servohydraulic test systems from MTS capable of running complex TMF tests. The capabilities of these systems, their accessories, and their digital controller and software architectures accommodate two unique aspects of Empa’s approach to TMF testing.
The first is the ability to reproduce the critical location stress/strain states of the component under actual turbine operating conditions. To do this, the test must switch from mechanical strain control to load control within the same cycle, which requires unprecedented flexibility. Advanced compensation techniques provide the precise servovalve control necessary to switch control modes. In addition, MTS TestSuite™ software allows operators to create highly customized wave shapes that incorporate the proper sequence of ramps and holds, as well as create a single, multi-segment cycle with two separate control modes.
MTS TestSuite software allows test designers to create customized wave shapes.
The second is the ability to superimpose high-frequency vibrations upon relatively low-frequency cyclic stress/strain conditions to simulate, for example, the gas bending loads on turbine aerofoils superimposed on cyclic centrifugal loading. The variation in gas pressure on the aerofoil is due to the cyclic interaction of moving and stationary blades, while the cyclic centrifugal loading is a consequence of the operating duty conditions of the turbine. Again, the control architecture of the MTS test solution delivers the processing speed and programming flexibility required to add these vibrations to the TMF test and create a highly realistic simulation.
CUSTOMER BENEFITS
The ultimate beneficiaries of Empa’s unique approach to TMF testing are manufacturers of gas and steam turbine components and structures, as well as the aircraft and power plant operators who deploy them. Because Empa can now generate and verify more accurate service life predictions that align with how turbines will actually be operated, manufacturers can develop and optimize new designs with confidence, and end-users can be equally confident the turbines will meet their requirements for performance, reliability, efficiency and safety.