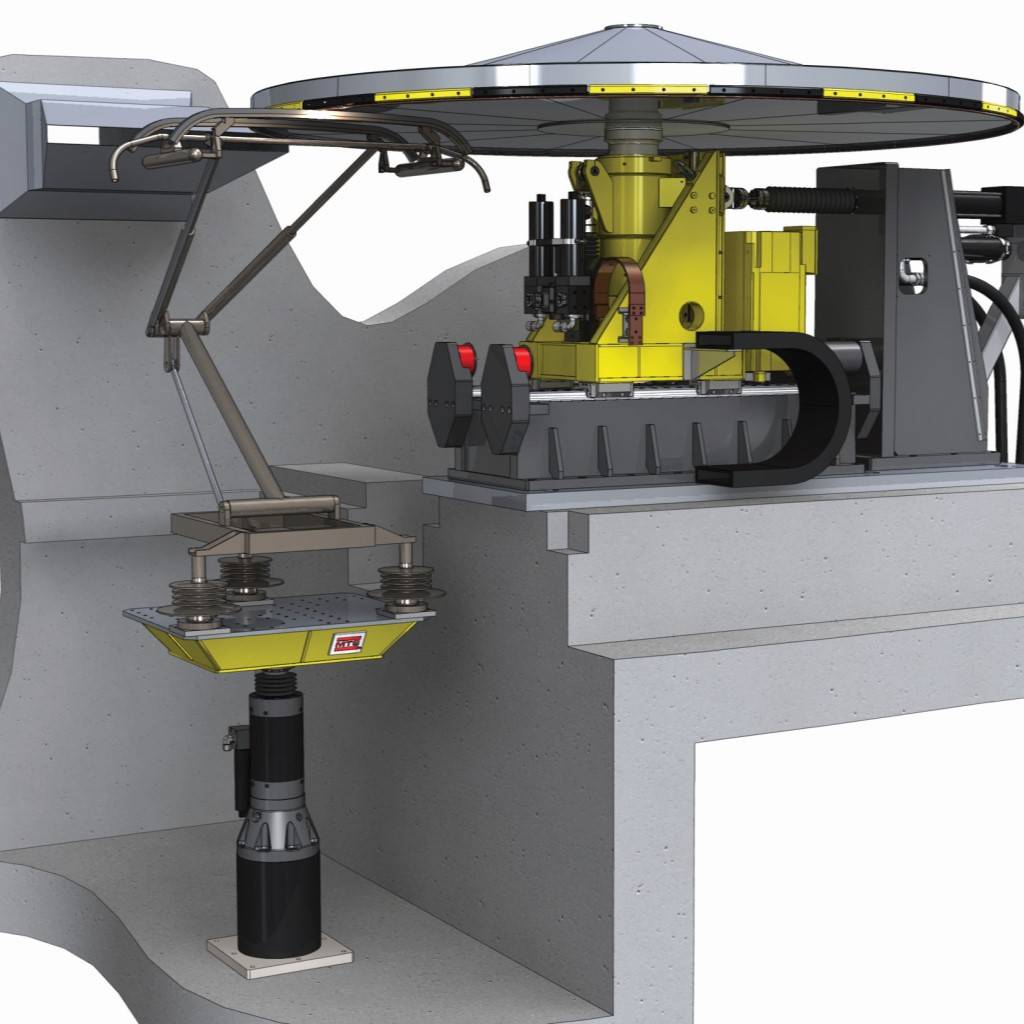
The point where the pantograph of an electric train makes contact with the trolley wire creates one of the most complex and challenging environments for rail component manufacturers and test engineers to understand, let alone predict and improve.
For trains to operate efficiently, the pantograph must maintain constant contact with trolley wires suspended from catenary systems. Yet these wires and their support structures exhibit different vertical stiffnesses along any given section. The catenary system zigzags in 30 to 100 meter intervals to prevent grooving. The force the pantograph applies to the wire must stay within a well-defined range (70N to 120N). If it is too low, loss of contact results in arcing, which not only causes the train to lose power but damages the trolley wire and the contact bar through etching and overheating. If the force is too high, the resulting friction wears down the wire and contact bar prematurely.
Delivering the right amount of force requires variable vertical motion. But when trains move at higher speeds, pantographs lose their ability to react appropriately. Even when the trolley wire is as flat as possible, it is only flat when it hangs undisturbed. When the pantograph lifts the wire, the resulting deformation creates a wave. If there is too much uplift, the pantograph creates a much larger waveform that causes contact problems for the next pantograph coming down the line.
Solving these problems is critical for the fast-growing global high-speed rail industry. Rail passengers demand fast, dependable service, but as rail cars run faster and faster, current pantograph technology puts speed and service quality at risk. In a worst-case scenario, a single worn trolley wire could crack and break, causing a failure that renders thousands of trips cancelled or rerouted while technicians complete the expensive, time-consuming process of replacing damaged wire.
RECREATING COMPLEX INTERACTIONS
From a testing perspective, the primary challenge is how to recreate the complex and dynamic pantograph operating environment in the lab. The pantograph is traveling at speeds up to 350 kph (220 mph) today, with ongoing development pushing that upper limit higher. The pantograph is also carrying enough power to accelerate hundreds of tons of rail cars to top speeds through a very small contact area. And as we’ve demonstrated, the physical components interact in a sliding environment with high longitudinal motion and varying degrees of pressure.
Traditional pantograph studies involve a basic spin/slide test, in which the trolley wire is attached to a spinning disc that is one or two meters in diameter. The test specimen is a piece of carbon or other contact material made to resemble the pantograph shoe. The specimen is pushed against the spinning wire and slid back and forth. These tests are valuable for characterizing wear effects on different materials, but they do not replicate the contact dynamics that occur with the actual system in service.
A new system developed by MTS Systems Corp. provides a more complete simulation of the pantograph environment. It enables manufacturers and test engineers to study pantograph and trolley wire dynamics with greater accuracy and precision. It is designed to help test groups measure off-line ratio (the time when contact is reduced), resistance of contact, power factor, arcing time and intensity, and temperature of the contact bars. It also enables test groups to perform real-time measurement of wear and profile change of the wire and contact bar.
By enabling these measurements, the system can be used to gain a better understanding of how new pantograph designs for high-speed rail will perform. Test engineers can perform effective studies of contact loss and related effects, contact resistance and arcing time, temperature of the arc and contact bar, and wear effects on the trolley wire and contact bar. Ultimately, test data will help manufacturers develop passive or active pantograph suspension systems that increase dynamic tracking of trolley wire motion, resulting in better contact while minimizing wear on the contact system.
TEST SYSTEM CAPABILITIES
This new system can closely replicate pantograph and catenary system dynamics for many different kinds of operating conditions rail cars experience around the world.
It simulates train speeds by mounting the trolley wire at the bottom edge of a 4m-diameter spinning disc that runs at operating speeds up to 550 kph. The disc can be programmed to simulate variable speeds as well as recreate real train speed time histories. To simulate the vertical displacement or stiffness of the trolley wire, the disc is mounted on motion carriage that is programmed to replay vertical displacement time history or simulate stiffness time history of the trolley wire. The carriage also moves in a zigzag motion to simulate the motion of the trolley wire over the track. The complete pantograph is mounted to a vibration table that simulates vertical motion of the rail car as it runs down the rail. To complete the simulation, the system can transmit up to 2,000 amps across the contact point. High-speed airflow is directed at the contact area to provide cooling and simulate the pantograph passing under the wire. And an instrumentation carriage follows the wire’s zigzag motion to provide real-time monitoring of wear on the contact bar and trolley wire.
The programmability of the individual components is what allows this test system to create more realistic simulations of actual pantograph and trolley wire interactions. Multiple test modes enable test engineers to play out actual time histories as well as vary all of the available test channels, including: wire vertical displacement, wire horizontal (zigzag) displacement, wire velocity, pantograph vertical motion, pantograph lift/drop, current flow and cooling air. The system can also provide a highly repeatable test scenario, allowing detailed measurements of small differences in test article design. These measurements allow engineering decisions and design changes to be made with greater confidence in how the components will perform in actual service environments.
During parametric tests, the disc is moved in a repeated cycle while different parameters are changed. For example, the disc can be programmed to maintain a constant vertical position, and then increasingly large displacements can be introduced and detailed studies made of the effect on contact performance. Synthetic data reproduction involves predicting trolley wire displacement history based on a digital model, with advanced signal processing software (such as MTS Remote Parameter Control, or RPC) used to achieve the desired disc motion history.
Finally, with real time history playback, trolley wire displacement is measured on-track and the software is used to recreate the time history of wire motion. The time base of the playback can be changed to simulate different train speeds, and short sections of actual operation can be connected and repeated to produce a drive file for the desired total distance.
MONITORING AND MEASUREMENT
One of the ways this test system enhances the ability of test engineers is by enabling the measurement of many factors that are nearly impossible to measure in “real world” circumstances, and to measure them in a standardized, repeatable way.
To do this, the system includes extensive test monitoring instrumentation. These include load measurement on the contact bars, contact load and drag load. Vertical acceleration is measured at each end of each contact bar, which allows monitoring of dynamic contact bar motion as it responds to the disturbances of the rail car and contact wire. Temperatures are monitored with transducers mounted to the back of the contact bar and a thermal imaging camera with recording capability. Laser scanners record the profile of the wire and contact bar for wear studies. Wire travel distance is recorded automatically. Current and voltage measurement are measured on both the input and output side of the contact point, allowing detailed monitoring of resistance, power factor and off-line time. Arcing time and intensity are optically monitored. All data is time-synchronized for detailed studies of specific instances or operational conditions.
Machine monitoring channels are also extensive. They include the main shaft bearings (vertical and horizontal acceleration as well as temperature), actuator loads and displacements, activity of the automatic lubrication system, pantograph lift/drop state, hydraulics and drive motor. Monitoring limits (including warning and shut-down levels) can be set on any data channel, giving test engineers precise control of system operation. These limit detectors, combined with the control and monitoring systems, enable automatic testing without the need for operators to monitor the machine continuously.
These capabilities can make a big difference for pantograph and train manufacturers. Measuring the many parameters of contact dynamics on a running pantograph, for example, is now a realistic proposition for test teams. In the past, this was an extremely difficult and impractical problem to solve due to poor repeatability and limited control. Today, more accurate simulations set the stage for more valuable insights. For example, test groups can perform more accurate calculations of power factor and loss, which have a direct effect on operating costs.
This system is part of the next wave of pantograph testing technologies that are changing the way rail test teams around the world conduct research. The market challenge is intense as trains run faster, rail lines expand into more regions and global ridership grows. Manufacturers will be able to meet the challenge with this and other test systems that enable test teams to achieve much more realistic simulations of complex pantograph and catenary system dynamics in the lab. With a more accurate and detailed understanding of the pantograph’s response in the operating environment, product designers will gain the insight they need to develop more efficient, reliable, high-performance pantographs.
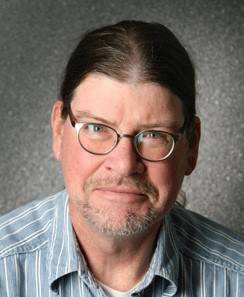